Fuel Cells
Fuel cell (FC) science and technology cut across multiple disciplines, including materials science, chemistry, electrochemistry, interfacial science, mechanical engineering and catalysis. The fuel cell (FC) is not a new invention, because its principle dates back to 1838. The question is not if FCs will play an important role in the future sustainable (carbon free) and energy efficient energy system, rather the question is when. The IEA (International Energy Agency) predicts FCs to be a significant part of the electricity generating market as well as for vehicle propulsion with significant market fractions in the long term future.
To reach commercialization the cell/system production cost (comparative to other technologies) must be decreased and the lifetime increased. It is not an exaggeration to say that almost all physical and chemical problems introduced in FCs have multiple scales in nature. The particle size in SOFC functional materials is in the sub‐micron scale, the three‐phase boundary (TPB) structure, design and catalytic activity are in the microscale and the cell/stack design is in the macroscale. The morphology and properties of these scales are important for the performance of the FC. It means that the sciences at a microscopic scale are critical to the performance at a macroscopic (system) scale. The broad range of length scales brings about an equally wide range of time scales, with chemical bond vibrations occurring over tens of femtoseconds and, at the other extreme, heating up a stack taking hours. The internal reforming and electrochemical reaction rates are dependent on the local microscopic material structure, catalytic activity, temperature and the concentrations. The ion and electron distributions are affected by the local temperature, the concentrations and also the porous material structure. The different polarizations depend on temperature, concentrations, porous material structure/design and catalytic activity. The above mentioned relationships, among several others, between the physical parameters and phenomena within a FC show the importance of the couplings between them, i.e., the reason why multiscale and multiphysics modeling and simulations are necessary and needed to understand the physical phenomena within FCs and for novel improvements of the FC overall performance. It should be noted that computational fluid dynamics (CFD) modeling makes it possible to reduce the amount of experiments needed for cell development, and only a limited amount of tests are then required to validate the accuracy of the models.
Our research in the field of fuel cells concerns analysis of heat and mass transfer and other transport phenomena in solid oxide fuel cells (SOFCs) and polymer electrolyte fuel cells (PEFCs). Numerical calculation methods have been used to predict various transport processes in flow channels in fuel cells; the electrochemical reactions in fuel cells, and their effects on energy and mass balances. The current research interests are focused on comprehensive understanding of chemical reactions and nano-/micro-structured porous material effects on various transport processes in various components of fuel cell systems.
Martin Andersson is representing Sweden in two IEA (International Energy Agency) Technology Collaboration Programme Advance Fuel Cells Annexes.
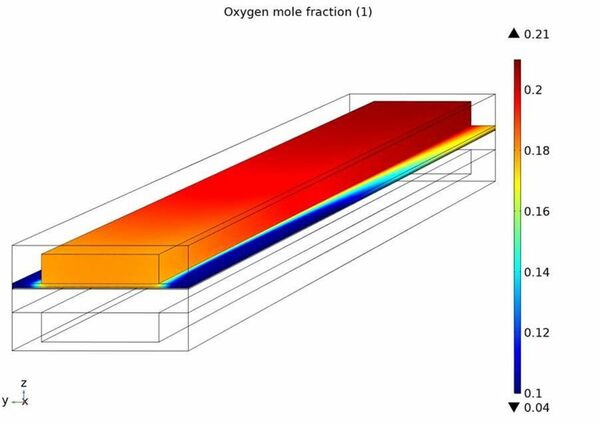